Seismic Data Acquisition
Seismic data acquisition is basically generation of seismic waves and recording them, using seismic sensors (or receivers) called geophones.
It consist of following basic components – acquisition geometry, seismograph (recording instrument), seismic source, and seismic receivers (geophones or hydrophones).
How Seismic Data is Recorded?
Source generate a seismic waves and seismic receivers record them on the surface, which are placed in a specified location or defined acquisition geometry.
Seismic data is used to collect information about the subsurface geological structures by measuring the reflection and refraction of seismic waves.
Generation of Seismic Wave
When seismic source is triggered energy is released instantly, it creates a high pressure wave that rapidly expand outward. This high pressure wave is followed by a low pressure area, or vaccum near the source point, a high energy sound waves are generated.
Seismic Sources
The various type of seismic sources are used to generate controlled seismic energy or seismic waves.
Here are some examples of seismic sources:
Explosives Source: In land data acquisition, explosives is most commonly used seismic source, which produce high-energy shock waves.
Vibroseis Trucks: it uses a vibrating plate to create controlled vibrations, used in land seismic data acquisition, particularly in desert are where sand are prominent on the surface.
Air Guns: Air guns, release compressed air bubbles into the water to generate sound waves in marine environments.
Wave Propagations
The seismic waves generated from triggered source, are travel through the Earth’s subsurface, reflecting and refracting off through the various geological layers. The behaviour of these waves are influenced by the properties of the materials they encounter.
There are two types of seismic wave – body waves and surface waves
Body wave (P & S Wave) – travels through the interior of the earth (travel inside the material body)
- Primary Wave (P-waves) – are compressional waves that are longitudinal in nature. They move faster and are the first to be detected by the receivers on the surface.
- Secondary Wave (S-waves) – are shear waves that are transverse in nature. They move slower than p-waves and are detected by the receivers on the surface after them.
Surface wave – travel across the surface of the earth (travel across the surface of material body).
In exploration seismology, the body waves (P & S-waves) are used to gather information about subsurface geological structures, helping geophysicists to identify and characterize potential resource deposits.
Seismic Receivers
Seismic Receivers: Seismic waves are recorded by receivers placed on the surface in a defined geometric patterns.
Type of seismic receivers:
- Geophones: Used on land to detect ground motion.
- Hydrophones: Used in marine environments to detect pressure wave.
- Seismometers: Highly sensitive instruments that can detect a wide range of seismic waves, often used in earthquake monitoring.
These sensors are deployed in a particular geometric pattern across the survey area to record the seismic waves.
Seismic Instrument (Seismograph)
Seismic data recording systems, are used to collect and store the data from the geophones on land. It has capability to record multiple channels for simultaneously in synchronize manners, and are equipped with various settings and parameters to optimize data quality.
Seismic Data Recording in Field
Seismic source generate waves, which travel through the earth, and reflected seismic waves are recorded by the receivers placed on the surface.
Synchronised Recording
In order to record seismic data in controlled manner, we use synchronise data recording it has 3-key components (Encoder-Communication-Decoder) is used to synchronise the seismic source activation, and recording in micro-seconds accuracy. An arial communication system is used to synchronise seismic data recording.
Observer enable fire command from Encoder at recording instrument, immediately data recording is started and signal is communicated to the decoder located near shot hole, it decodes signal received from the instrument, and a time break is recorded known as Decode Time Break (DTB).
The decoder send high voltage (400V) to the detonator terminal which is a closed-circuit.
The detonator triggerd, the explosion take place, and the circuit is breaken, hence voltage is drop drastically, this sudden drop in voltage is recorded at the decoder, which confirms that shot is blasted, and a time new break is recorded known as CTB (confirmation time break). This information is send to the instrument.
Once shot is blasted, a seismic energy released and propagates in all directions, and the first arrivals of seismic wave hit to the geophone planted near the shot hole (also known as uphole geophone), and uphole time is recorded.
The direct arrivals of seismic waves are recorded first in the shot record and the reflected arrivals are recored at later time in shots the shot gathers.
This process of synchronise seismic recording is repeated for every recorded shots in the field. This ensures that data is collected consistently and accurately across the survey area.
Auxiliary (Aux) channels
Auxiliary (Aux) channels in seismic data recording help capture following information in the field records:
ITB (Initial Time Break)
It is the time at which the is fired command from instrument is initiated to activate seismic energy source.
FTB (Final Time Break)
It marks the end of the seismic energy source’s active phase.
Uphole Time (Up)
The time taken by seismic waves to travel from the seismic source to the surface, where an uphole geophone is placed. This uphole time is used to determine the velocity of seismic waves in the near-surface.
Dynamic Range of Seismic System
The dynamic range of a seismic data recording system refers to its ability to accurately capture signals of varying amplitudes, from the weakest to the strongest, without any loss of information.
For example 24-bit seismic data recording system have dynamic range 144.492dB.
In terms of signal amplitude it is calculated in dB by using logarithms to base 10.
Dynamic range of a Q-bit system:
DR = 20 log10 (2bit depth) = 20 log10 (2Q)
Dynamic range of a 1-bit system:
DR (in dB) = 20 log10 (21) = 6.02dB
Dynamic range of a 24-bit system:
Dynamic Range (in dB) = 20 log10 (224)
Dynamic Range (in dB) = 20 log10 (16,777,216)
≈ 144.49439 dB
So, the dynamic range of a 24-bit system is approximately ≈144dB.
Dynamic range of different recording systems and seismic recording sensors may vary.
Quick Calculation of Dynamic Range
The dynamic range of a Q-bit system = (6.02*Q + 1.76 correction factor) dB.
So, for 24-bit system = (6.02*24 + 1.76) dB. = 146.24dB.
A correction factor of 1.76 is included, which accounts for the quantization noise introduced by the digital representation of the analog signal.
The dynamic range of a trace display is controlled by gain settings.
Acquisition Parameters
Pre-Amplification Gain Settings
Pre-amplification gain amplify the weak seismic signal, it aim to boost weak trace and improve signal-to-noise ratio (S/N ratio). Most practical and commonly used pre-amplification gains are 6dB or 12dB. However, it is always decided after experimental results.
Filters
The main purpose of the filters are to remove unwanted noise or frequencies from the recorded seismic data. These days no filters are applied in raw data, except high-cut, because we can easily filter noise frequency later while data processing. So, raw data is recorded without filter application.
High-cut filter (anti-alias filter) is applied to avoid aliasing in the recorded data.
Sampling Rate
The rate at which seismic data is sampled, usually specified in millisecond (ms), the most of the seismic data utilised for hydrocarbon exploration is recorded with 2ms sampling rate. It is user specified parameter in the recording instrument and this parameter remain constant for entire project.
Recording Format
The seismic data is recorded and stored in formats, such as SEG-Y or SEG-D depends on the data recording instrument, each instrument have it’s own format.
Acquisition Geometry
Survey Design
The seismic survey geometry design is very crucial part of seismic data acquisition. The arrangement of sources and receivers is planned based on the objectives of the survey, such as target depth also known as depth of investigation.
2D Geometry
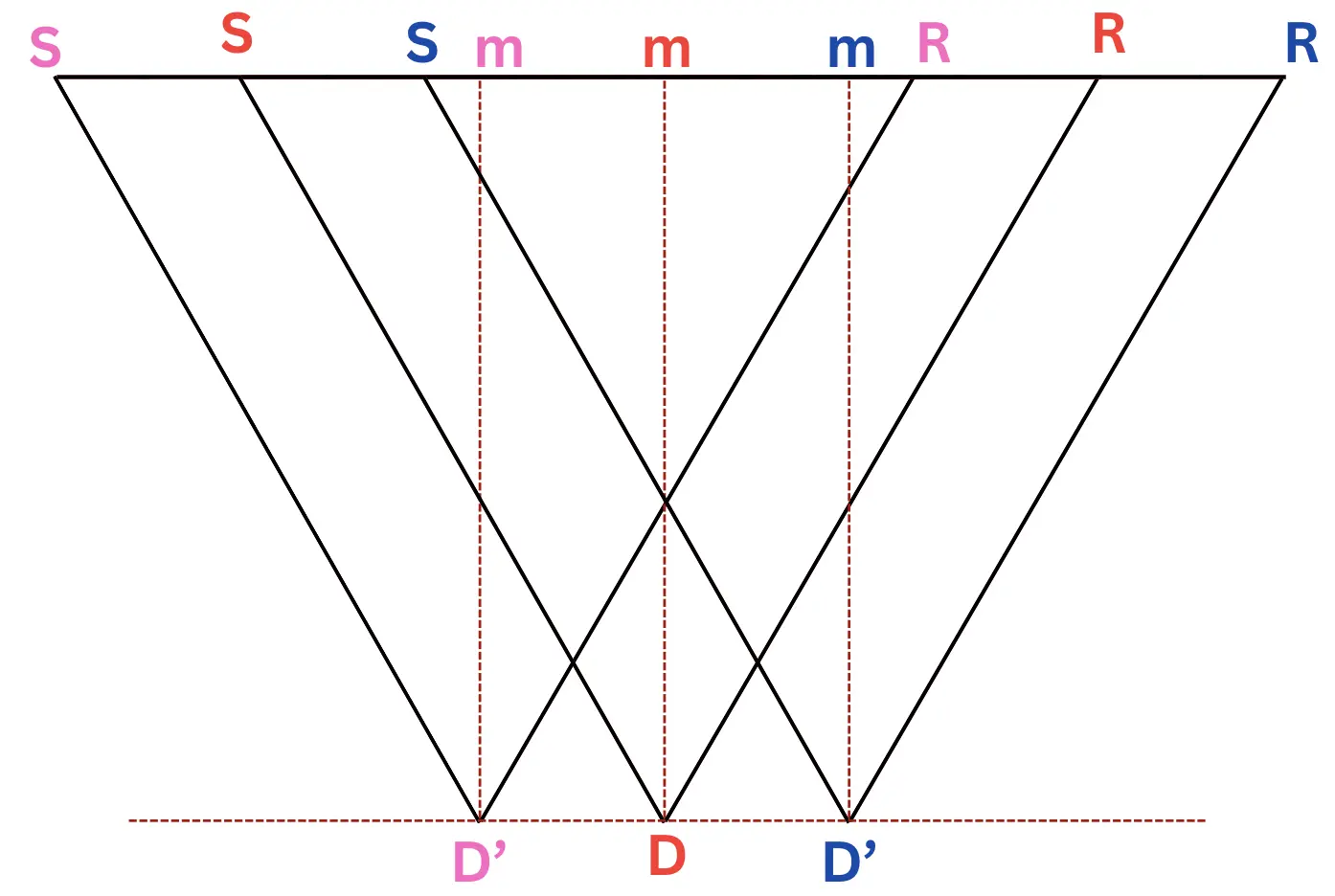
Imagine a equilateral triangle, place source, receiver and cdp at each corner of the triangle, remember the triangle is in inverted position. This indicate the recording geometry for 2D data. Move this inverted equilateral triangle to record entire seismic line.
The seismic reflection is recorded at 30 degree angle (near-vertical) using geometry of equilateral triangle optimises the acquisition geometry for capturing reflections from various depth and subsurface structures.
Seismic Fold
In seismic reflection surveys, the fold refers to the number of times a particular subsurface point is mapped by seismic waves. In 3D seismic survey fold is calculated by summing the number of traces falls within the bin.
At smaller angle, reflections from both shallow and deep layers are effectively recorded, providing a balanced view of the subsurface, whereas the reflections at higher angle cause signal attenuation due to increased travel distance leading to poorer image quality.
QC of Seismic Data Recording
Data QC is critical step to ensure the quality of the seismic data recorded in the field. Ensure that the planned source and receiver locations match the actual field setup and deviations if any is properly recorded. QC for recording precise coordinates and elevation for source and receiver locations in very important.
Calibration for Recording Instrument and other Field Equipments
Tab test shall be conducted before deploying equipments in the field to maintain accuracy of recorded seismic data.
QC for Recorded data (Signal Quality)
Monitor the amplitude, frequency, and coherence of seismic signals to ensure the good quality seismic data is recorded.
Setting Quality Standards (Thresh-hold Parameters)
You have to set quality standard for noise and other factors, which can potentially degrade seismic data such as noise monitoring parameters and thresh hold to allow, you can identify and mitigate various type of noises such as equipment interference, cultural noise, ambient noise, traffic or highway noise, and noise due to industry or machinery.
Receiver Plantation and Functionality
Ensuring that all geophones or receivers are planted properly with good coupling and working correctly and recording data as expected.
Source Performance
Verify that the seismic source is generating the expected energy levels and waveforms or pulse shape.
Parameter Checks
Verify acquisition parameters such as sample rate, recording length, instrument time-break, and quality control thresh-holds.
Coverage & Recovery
Ensure adequate data coverage and recovery shots to compensate for any gaps or missing data for the planned survey geometry.
Learn More About Seismic
Click 👉 Seismic Data Acquisition
Click 👉 Seismic Survey Geometry
Click 👉 Seismic Data Processing
Click 👉 Seismic Depth Imaging
Click 👉 Seismic Data Interpretations